H. ZHANG, B. ZHU, S. PARK, C. DOERR, M. AYDINLIK, J. GEYER, T.PFAU, G. PENDOCK, R. AROCA, F. LIU, C. RASMUSSEN, B. MIKKELSEN, P. I. BOREL, T. GEISLER, R. JENSEN, D. W. PECKHAM, R. LINGLE JR., D. VAIDYA, M. F. YAN, P. W. WISK, AND D. J.DIGIOVANNI
Acacia Communication, Inc., 3 Mill and Main Place, Suite 400, Maynard, MA 01754, USA 2
OFS, 19 Schoolhouse Road, Somerset, NJ 08873, USA *
Abstract: We demonstrate real-time transmission of 16 Tb/s (80x200Gb/s) over 1020km TeraWave ULL fiber with 170km span length using the world’s first 200Gb/s CFP2 module with a record low power consumption less than 0.1W/Gbps.
© 2018 Optical Society of America under the terms of the OSA Open Access Publishing Agreement
OCIS codes: (060.1660) Coherent communications; (060.2330) Fiber optics communications; (060.4510) Optical communications.
1. Introduction
The rise of social media and over-the-top video along with the adoption of mobile broadband have driven fast traffic growth and shifting traffic patterns in the metro and regional networks. For metro applications, the transmission reaches have to be above 50 km for metro/access and below 1000 km for metro/regional applications. Different from long-haul (>2000 km) transmission systems which heavily focus on the reach and the performance, the metro application calls for compact, scalable, cost-efficient, and easy-to-use digital coherent transport solutions [1,2] as shown in Fig. 1. These requirements are met with pluggable CFP2 transceiver modules (“digital coherent optics”) that include all optics and digital signal processing to support plug-and-play coherent transmission with flexible capacity and spectral efficiency (SE).
In order to achieve the capacity and cost-per-bit targets of metro/regional systems, it is essential to be able to increase the data rates from 100Gb/s to 200Gb/s while maintaining sufficient robustness to narrow optical filtering resulting from cascaded reconfigurable optical add-drop multiplexers (ROADM) on 37.5 and 50 GHz grids. To achieve this goal, 200Gb/s CFP2 should support high modulation formats like 8QAM and 16QAM. However, the lower OSNR sensitivity and increased susceptibility to distortions of higher modulation order pose additional challenges to a CFP2, which is constrained by low power consumption and small footprint requirements. To address this challenge, careful power optimization of DSP algorithm and a good trade-off between DAC/ADC ENOB and performance are necessary. Also, a novel design of RF driver architecture further reduces the power dissipation.
In this paper we report real-time transmission of 200Gb/s CFP2 signals over 1020 km TeraWave ULL fiber using a span length of 170km, much longer than typical metro/regional spans. This length was chosen to stress the OSNR budget while showing achievable amplifier spacing with ultra-low loss fiber. We demonstrate transmission of 80 x 200Gb/s polarization multiplexed (PM) 8QAM and 16QAM channels on a 50 GHz grid with 2.8dB and 1.2dB Q2-factor margin, respectively. We further confirm that there is only 0.1dB Q2-factor penalty for 200Gb/s PM 16QAM signals at 37.5GHz grids compared with 50 GHz channel spacing. We also make a 19 hours’ long-term error free measurement for 200Gb/s PM-16QAM signals at 37.5GHz spacing over 1020km. We report <0.1 W per Gb/s power consumption, which is only 1/8 of an OIF compliant 100G coherent 5”x7” module [3]. To the best of our knowledge this is the first demonstration of real-time 200Gb/s transmission enabled by CFP2 modules and the lowest reported power consumption per bit for coherent transmission.
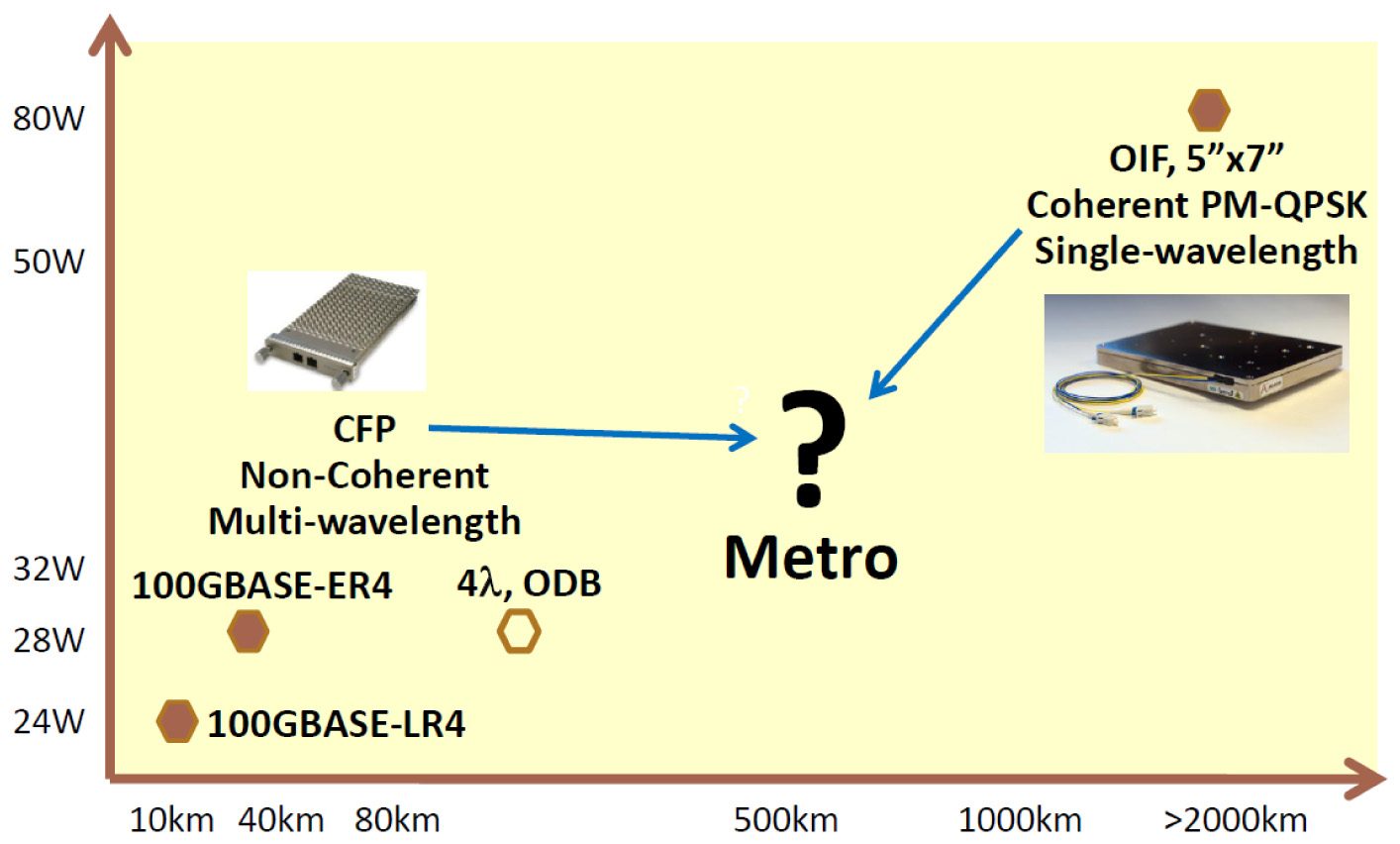
Fig. 1. Transceiver module landscape (Ref [2].).
2. CFP2 transceiver module
The CFP2 module used in this experiment is implemented in multi-source agreement (MSA) 107.5 mm x 41.5 mm form factor. It has twice the faceplate density of CFP-DCO. It integrates a tuneable narrow-linewidth laser, a single-chip silicon photonic integrated circuit for quad-parallel Mach-Zehnder modulators and polarization diversity 90° optical hybrid, trans-impedance amplifiers (TIA), RF drivers, and a DSP ASIC based on 16-nm CMOS technology. It supports 100Gb/s client data rate using 31.4Gbaud PM-QPSK and 200Gb/s client data rate using either 41.8Gbaud PM-8QAM or 31.4Gbaud PM-16QAM.
The ASIC includes four-channel DAC/ADC, a DSP engine which performs pulse shaping and pre-equalization on the transmitter side, and compensation of chromatic dispersion and polarization mode dispersion as well as clock and carrier recovery on the receiver side. One of the key features of the DSP is a soft-decision forward-error correction based on TurboProduct code designed specifically for ultra-low power consumption applications. With 15% overhead, it has up to 10.8dB net coding gain (NCG) for QPSK and 11.3dB NCG for 16QAM. Low-pow silicon photonic circuits [4] and RF drivers together with the carefully power optimized DSP [5,6] enable a total power consumption of the CFP2 module of less than 20W for 200Gb/s capacity.
Figure 2 shows the comparison of AC200 CFP2 product with other Acacia’s coherent transceivers including AC040, AC100 and AC400 in terms of watts per 100Gb/s. The improvement of CMOS technology from 40nm to 16nm together with the advanced optical/DSP design, which increase the modulation format from BPSK to 16QAM, enable to reduce the power consumption per 100Gb/s from 125W to 10W over 5 years. It is important to note that this roadmap will continue in both directions in the future. First, the date rate will be further increased to 400Gb/s and beyond. Second, the module has even smaller form factor like OSFP or DD-QSFP [7,8] and less power consumption thanks to the next generation of 7nm CMOS technology. The industry expects the 80km-plus 400ZR digital coherent optics module will consume around 15W [9], which results in only 3.75W per 100Gb/s.
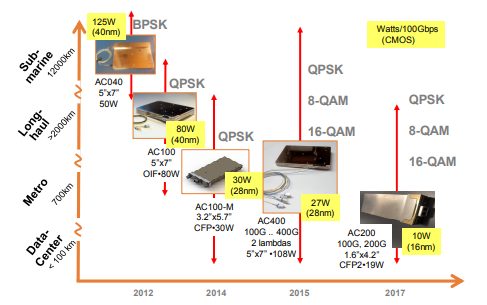
Fig. 2. Evolution in power and density per 100Gbps for coherent transceiver modules.
3. Experimental setup
The experimental set-up is shown in Fig. 3. The transmitters consist of one loading and one measurement path. For the measurement path, four 200Gb/s CFP2s (either 16QAM or 8QAM) with a root raised cosine (RRC) spectrum (ROF = 0.2) are set to adjacent channels and then combined together. The loading path is composed of 80 CW DFB lasers on 50GHz spacing from 1530.334 to 1561.826nm, and it is modulated by an I/Q modulator which is driven with 32Gbd 16QAM data waveform by a two-channel 64GS/s DAC using a test sequence generated from an off-line DSP. The polarization multiplexing is performed with passive components. The four 200Gb/s CFP2 measurement channels are combined with the loading path which is notch-filtered by a wavelength selective switch (WSS) prior to the combination. The combined 80 DWDM channels are sent to transmission fiber links with equal launch power. The transmission link consists of six 170km spans using OFS TeraWave ULL fiber which has Aeff of 125 um2 and average attenuation of 0.155dB/km at 1550nm.
The long spans are amplified by hybrid Raman/EDFAs. The average span loss including splices, connectors, WDM couplers and isolators for Raman pumps is 29dB. The average EDFA gain is about 16.3dB. The averaged on-off Raman gain is about 12.7dB which is enabled by three pump lasers at 1429nm, 1447nm, and 1465nm with a total pump power of 850mW.
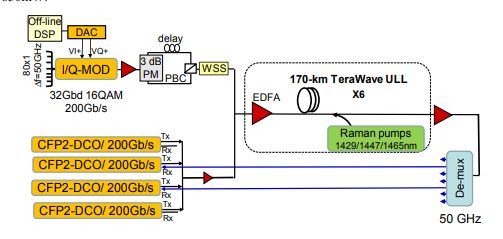
Fig. 3. Circulating loop setup for transmission experiments.
4. Experimental results and discussion
To test the 200Gb/s transmission performance as a function of OSNR for PM-8QAM on a 50GHz grid (4 bits/s/Hz SE) and PM-16QAM on a 37.5GHz grid (5.33 bits/s/Hz SE), we place the 4 CFP2 channels next to each other in the middle of the C-band. One of the middle CFP2 channels is selected by a 50GHz optical demux in front of the coherent receiver and the real-time BER is measured as a function of received OSNR by varying the EDFA output power and subsequently converted into Q2-factor.
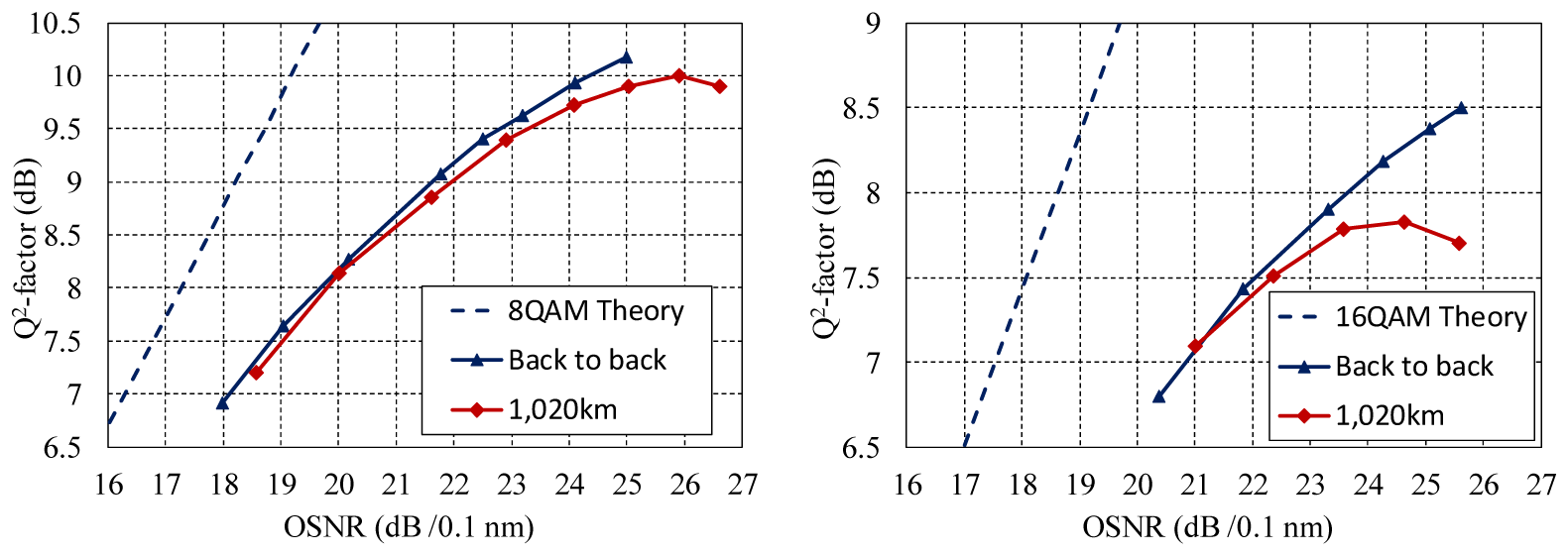
Fig. 4. PM-8QAM and PM-16QAM pre-emphasis measurement at 4 bits/s/Hz and 5.33 bits/s/Hz SE in back-to-back and transmission.
Figure 4 show the PM-8QAM and PM-16QAM performance vs. OSNR at 1545.7nm after 1020 km along with the noise loaded WDM back-to-back performance at 4 bits/s/Hz and 5.33 bits/s/Hz SE. At the error correction threshold (~6.6dB Q2-factor), we achieve a minimum required OSNR of 17.5dB/0.1nm and 20dB/0.1nm respectively. After transmission, the optimal OSNR is achieved at 25.8dB/0.1nm for PM-8QAM and 24.6 dB/0.1nm for PM16QAM. Also, the optimal power per channel is achieved at 1.5dBm for PM-8QAM and 0.3dBm for PM-16QAM. The difference of optimal power per channel is approximately equal to the ratio of symbol rate between PM-8QAM and PM-16QAM. This result indicates the optimal power density is the same for different modulation formats, which is consistent with the Gaussian Noise (GN) model for dispersion uncompensated systems [10]. For the same 200Gb/s net data rate, PM-8QAM at 50GHz channel spacing outperforms PM-16QAM at 37.5 GHz channel spacing by ~2dB Q2-factor at optimal power per channel.
To test the transmission performance as a function of the wavelength, we determine the Q2-factor from the measured real-time BER of the two middle channels among 4 consecutive CFP2 50GHz-spaced channels as they are moved across the C-band. The EDFA operates at 19.2dBm output power, which corresponds to an average power per channel of 0.2dBm launched into the transmission fiber. The average OSNR across 80 channels is 23.7dB/0.1nm. The result of this measurement is shown in Fig. 5. It shows that PM-8QAM and PM-16QAM have an average of 2.8 dB and 1.2dB Q2-factor margin above the FEC threshold (~6.6dB) respectively.
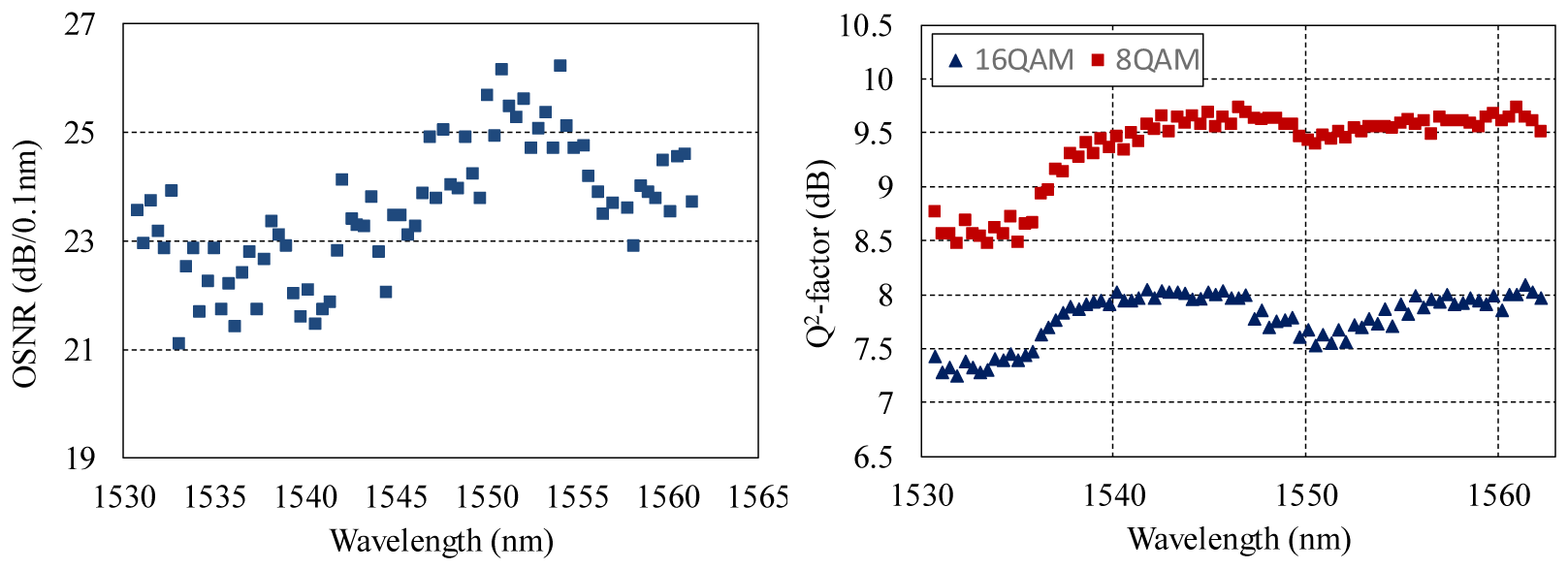
Fig. 5. Measured OSNR and Q2-factor performance across 80 channels at 50GHz channel spacing after 1020 km.
Due to the larger symbol rate, PM-8QAM will be more sensitive than PM-16QAM to filtering and misalignment of the transmit laser and the center frequency of the filters. However, Fig. 6 shows that there is only 0.5dB Q2-factor degradation after 6GHz detuning although the received spectrum after 1020km transmission is asymmetric after passing through a 50GHz optical demuxer in front of the coherent receiver.
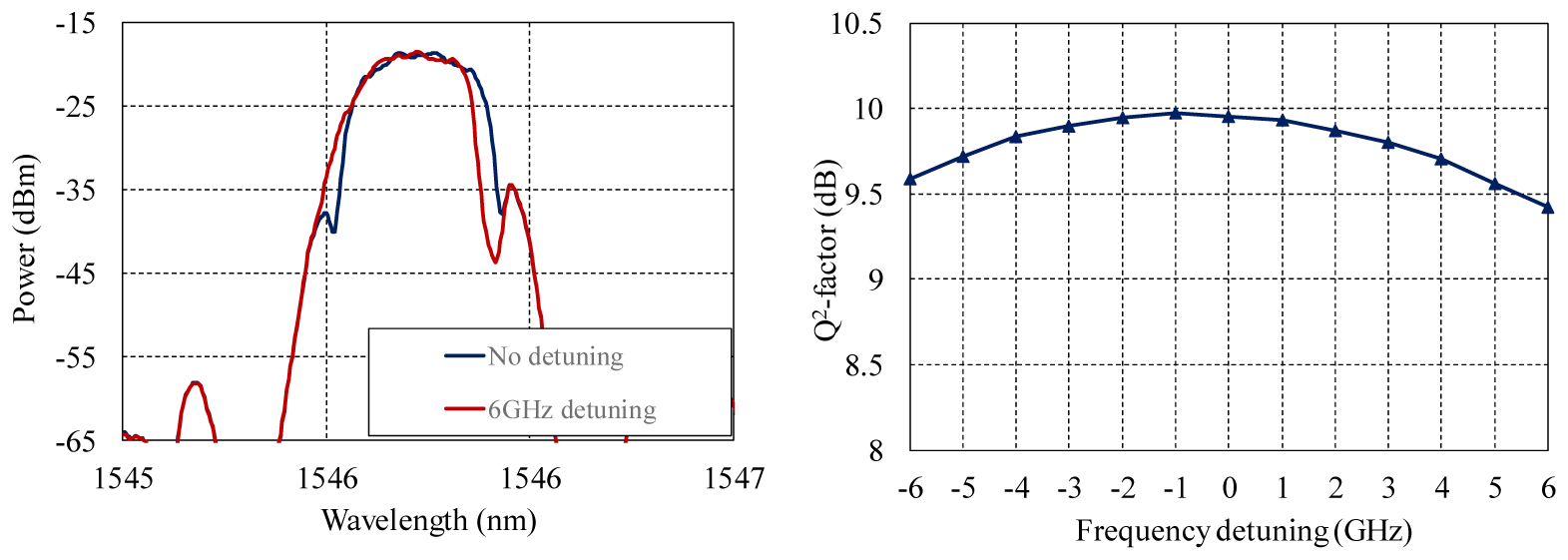
Fig. 6. Left: Optical spectrum of PM-8QAM after 50GHz demuxer w/o frequency detuning. Right: Performance penalty with frequency detuning after 1020km.
Figure 7 shows the result of the 19 hours long-term performance measurement of PM16QAM at 37.5GHz channel spacing after 1020km transmission. The measurement samples are collected at 2 second interval. All transmitted data are decoded without error. We see only 0.1dB Q2-factor degradation compared with 50GHz channel spacing. It implies that WDM crosstalk penalty is small. We also confirm that the CFP2 module power consumption is less than 20W.
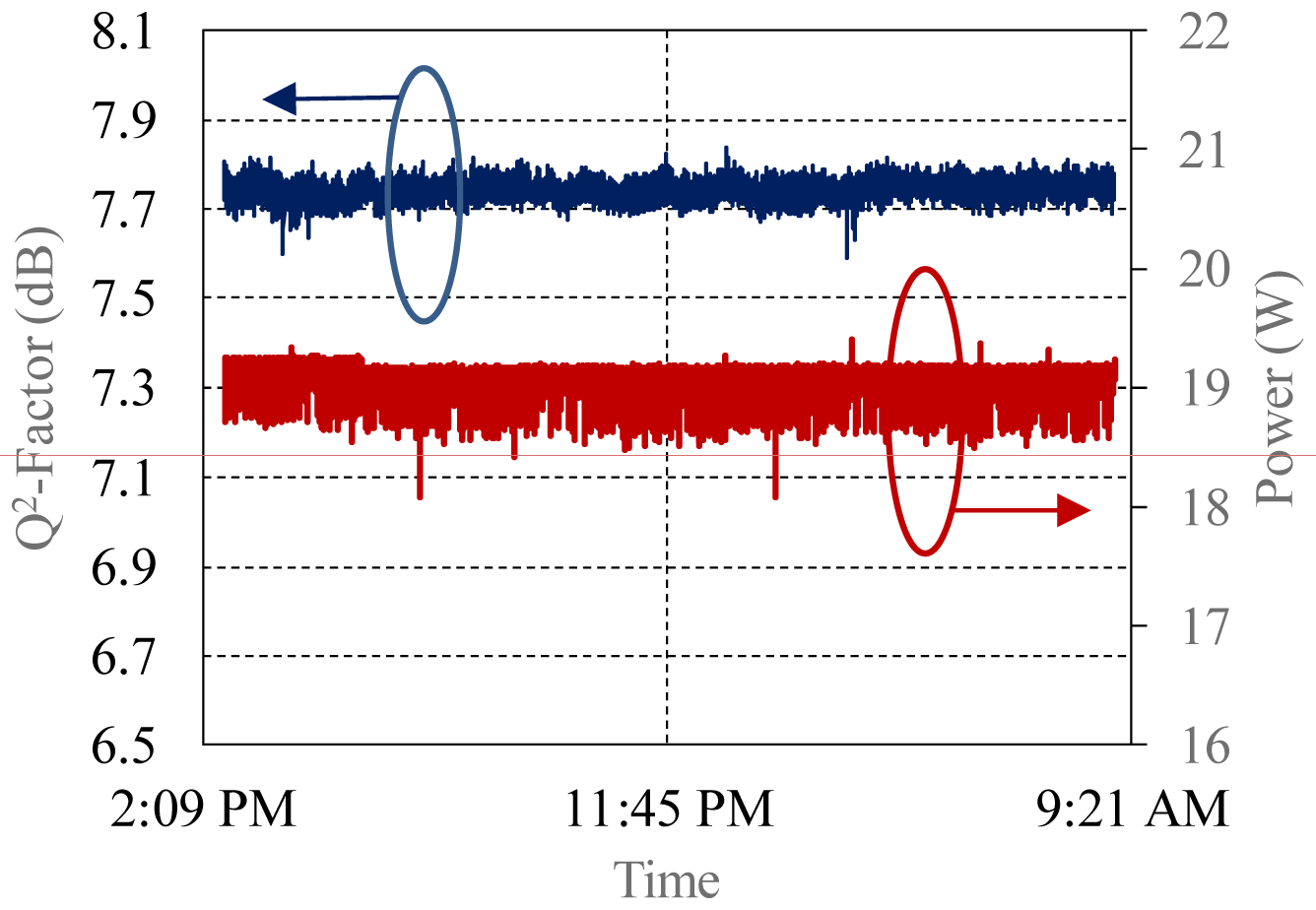
Fig. 7. Long term measurement of PM-16QAM at 37.5 GHz channel spacing.
5. Conclusions
Relying on pluggable CFP2 transceiver modules, we demonstrate successful 200Gb/s real-time transmission of 80 PM-8QAM and PM-16QAM channels at 4 bits/s/Hz spectral efficiency over 1020 km distance using very long 170km spans of TeraWave ULL fiber showing 2.8dB and 1.2dB Q2-margin, respectively. We make a long-term measurement of PM-16QAM at 5.33 bits/s/Hz spectral efficiency showing the potential for further increase of the system capacity. At less than 0.1W/Gbps, we achieve by far the lowest reported power consumption per bit for coherent optical transceivers.
Acknowledgement
We thank many colleagues at Acacia who were essential in the development of the CFP2.
References and links
- http://www.tmcnet.com/tmc/whitepapers/documents/whitepapers/2013/9378-bell-labs-metro-network-trafficgrowth-an-architecture.pdf
- http://www.ecocexhibition.com/sites/default/files/file/Market%20Focus%20Presentations%202012/Acacia%20ECOC%202012.pdf
- http://www.oiforum.com/public/documents/OIF-MSA-100GLH-EM-01.0.pdf
- C. R. Doerr, “Photonic Integrated Circuits for High-Speed Communications,” presented at the 2010 Conference on Lasers and Electro-Optics (CLEO) and Quantum Electronics and Laser Conference (QELS), San Jose, California, 16–21 May 2010, CFE3.
- J. C. Geyer, C. Rasmussen, B. Sha, T. Nielsen, and M. Givehchi, “Power Efficient Coherent Transceivers,” presented at the 42nd European Conference on Optical Communication (ECOC), Dusseldorf, Germany, 18–22 Sept. 2016, P109.
- C. Rasmussen, “Power and Reach Trade-offs Increasing the Optical Channel Rate Through Higher Baud Rate and Modulation Order,” in Optical Fiber Communication Conference, OSA Technical Digest (online) (Optical Society of America, 2017), paper Th3G.1.
- http://www.lightwaveonline.com/articles/2016/11/osfp-msa-targets-400-gbps-optical-transceiver-module.html
- http://www.lightwaveonline.com/articles/2016/03/qsfp-dd-msa-targets-200g-400g-optical-transceivers.html
- http://www.gazettabyte.com/home/2017/6/22/the-oifs-400zr-coherent-interface-starts-to-take-shape.html
- P. Poggiolini, “The GN Model of Non-Linear Propagation in Uncompensated Coherent Optical Systems,” J. Lightwave Tech. 30(24), 3857–3879 (2012).